Ir Spectrum

In the realm of analytical chemistry, few techniques rival the power and versatility of infrared (IR) spectroscopy. As a non-destructive method for identifying and quantifying chemical substances, IR spectroscopy has become an indispensable tool in various fields, including organic chemistry, biochemistry, and materials science. At its core, IR spectroscopy relies on the interaction between infrared radiation and matter, providing a unique molecular fingerprint that enables the identification and characterization of compounds.
The Fundamentals of Infrared Radiation
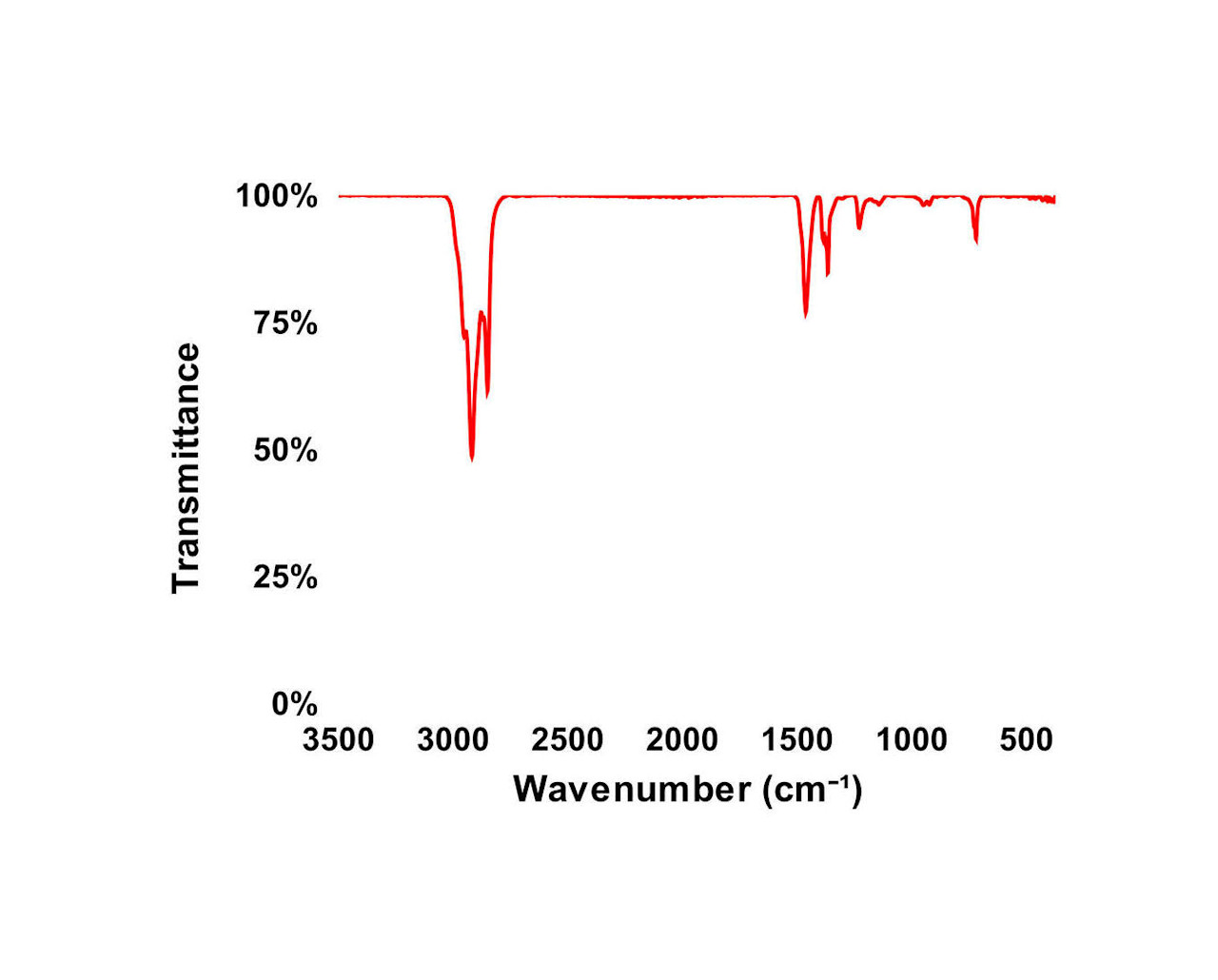
Infrared radiation, a subset of the electromagnetic spectrum, spans wavelengths ranging from approximately 750 nm to 1 mm. This region is further divided into three sub-regions: near-infrared (NIR, 750 nm – 2.5 μm), mid-infrared (MIR, 2.5 μm – 50 μm), and far-infrared (FIR, 50 μm – 1 mm). The MIR region, in particular, is of significant interest in IR spectroscopy, as it corresponds to the vibrational frequencies of many chemical bonds.
When a molecule absorbs infrared radiation, it undergoes a transition from its ground state to an excited vibrational state. This absorption occurs at specific wavelengths, which are characteristic of the molecule's chemical structure. The resulting spectrum, known as an IR spectrum, serves as a unique molecular signature, allowing for the identification and quantification of compounds.
Vibrational Modes and Molecular Symmetry

The vibrational modes of a molecule are determined by its chemical structure and symmetry. According to the Hooke’s law approximation, the potential energy of a diatomic molecule can be modeled as a harmonic oscillator. In more complex molecules, the normal modes of vibration are linear combinations of the individual atomic displacements. The number of normal modes is given by the formula:
3N - 6 (for non-linear molecules) or 3N - 5 (for linear molecules)
where N is the number of atoms in the molecule. These normal modes can be categorized into different types, including stretching, bending, and torsional vibrations.
The symmetry of a molecule plays a crucial role in determining its vibrational spectrum. Symmetry operations, such as rotations and reflections, can reduce the number of observable vibrational modes, leading to selection rules that govern the allowed transitions.
IR Spectroscopy Techniques
There are several techniques used in IR spectroscopy, each with its own advantages and limitations. Some of the most common techniques include:
- Transmission IR Spectroscopy: In this technique, a sample is placed between two infrared-transparent windows, and the transmitted radiation is measured. This method is suitable for solid, liquid, and gas samples, but requires careful sample preparation to ensure uniformity and avoid scattering.
- Attenuated Total Reflectance (ATR) IR Spectroscopy: ATR-IR spectroscopy involves the use of an internal reflection element (IRE), typically made of a high-refractive-index material like zinc selenide or diamond. The sample is placed in contact with the IRE, and the evanescent wave generated at the interface is used to probe the sample. ATR-IR is particularly useful for analyzing solids, liquids, and pastes, as it minimizes the effects of scattering and does not require extensive sample preparation.
- Fourier Transform Infrared (FTIR) Spectroscopy: FTIR spectroscopy is a powerful technique that uses an interferometer to measure the infrared spectrum. By collecting an interferogram and performing a Fourier transform, the spectrum can be reconstructed with high resolution and sensitivity. FTIR is widely used in various applications, including chemical analysis, materials characterization, and biological research.
Applications of IR Spectroscopy
The versatility of IR spectroscopy has led to its widespread adoption in numerous fields. Some notable applications include:
- Organic Chemistry: IR spectroscopy is used to identify and characterize organic compounds, including functional groups, bond types, and molecular structures. It is also employed in reaction monitoring, product identification, and quality control.
- Biochemistry: In biochemistry, IR spectroscopy is used to study proteins, nucleic acids, and other biomolecules. It provides valuable information about secondary structures, conformational changes, and molecular interactions.
- Materials Science: IR spectroscopy is used to characterize materials, including polymers, ceramics, and composites. It can provide insights into material properties, such as crystallinity, molecular weight, and thermal stability.
- Environmental Analysis: IR spectroscopy is employed in environmental analysis to detect and quantify pollutants, such as pesticides, heavy metals, and volatile organic compounds (VOCs).
Advantages and Limitations of IR Spectroscopy
Like any analytical technique, IR spectroscopy has its advantages and limitations. Some of the key benefits include:
- Non-destructive analysis
- High sensitivity and selectivity
- Rapid analysis times
- Minimal sample preparation requirements
However, IR spectroscopy also has some limitations, such as:
- Limited sensitivity for certain compounds (e.g., inorganic species)
- Potential for interference from water vapor and other atmospheric components
- Difficulty in analyzing complex mixtures with overlapping spectra
Data Analysis and Interpretation
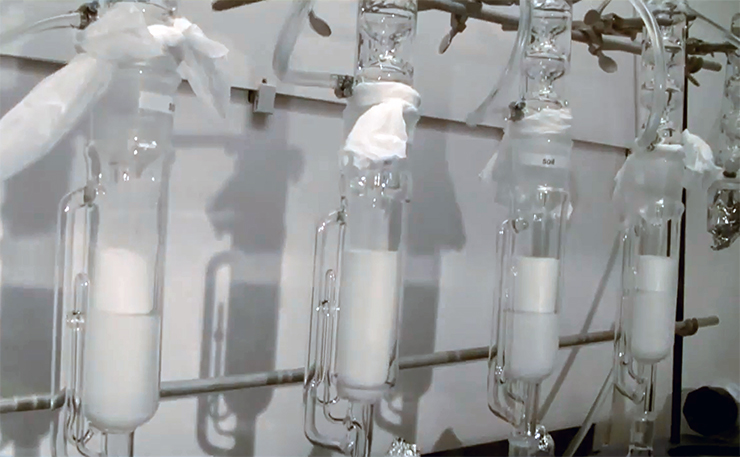
The interpretation of IR spectra requires a combination of theoretical knowledge, practical experience, and computational tools. Several databases, such as the NIST Chemistry WebBook and the SDBS (Spectral Database for Organic Compounds), provide reference spectra for thousands of compounds. Additionally, software packages like OPUS and GRAM-X offer advanced data processing and analysis capabilities.
Steps for IR Spectrum Interpretation
- Identify the region of interest (e.g., functional group region: 4000-1500 cm-1)
- Locate the characteristic absorption bands and their corresponding wavenumbers
- Compare the observed bands with reference spectra or database entries
- Consider the molecular structure and symmetry to assign the observed bands to specific vibrational modes
- Use computational tools, such as density functional theory (DFT) calculations, to validate the assignments and refine the interpretation
Future Directions and Emerging Trends
The field of IR spectroscopy continues to evolve, driven by advances in technology, instrumentation, and data analysis. Some emerging trends include:
- High-Throughput IR Spectroscopy: The development of automated sampling systems and rapid data acquisition techniques enables high-throughput analysis, making IR spectroscopy more suitable for large-scale studies and industrial applications.
- Hyperspectral Imaging: Hyperspectral imaging combines IR spectroscopy with spatial resolution, allowing for the analysis of complex samples with varying chemical compositions. This technique has applications in fields like art conservation, forensic science, and medical diagnostics.
- Machine Learning and Artificial Intelligence: The integration of machine learning algorithms and artificial intelligence enables more accurate and efficient data analysis, facilitating the interpretation of complex IR spectra and the discovery of new chemical insights.
What is the difference between FTIR and dispersive IR spectroscopy?
+FTIR (Fourier Transform Infrared) spectroscopy uses an interferometer to collect an interferogram, which is then Fourier-transformed to obtain the spectrum. In contrast, dispersive IR spectroscopy uses a monochromator to separate the infrared radiation into its component wavelengths. FTIR offers several advantages, including higher sensitivity, faster data acquisition, and improved signal-to-noise ratio.
How does temperature affect IR spectra?
+Temperature can significantly affect IR spectra, particularly for molecules with low-frequency vibrational modes. As temperature increases, the population of excited vibrational states grows, leading to changes in the intensity and shape of absorption bands. Additionally, temperature can influence the sample's physical state, affecting its interaction with infrared radiation.
What are the main sources of error in IR spectroscopy?
+The main sources of error in IR spectroscopy include: scattering effects, particularly for solid samples; atmospheric interference from water vapor and carbon dioxide; and instrumental artifacts, such as detector noise and optical misalignment. Careful sample preparation, proper instrument calibration, and appropriate data processing techniques can help minimize these errors.
How is IR spectroscopy used in protein analysis?
+IR spectroscopy is used in protein analysis to study secondary structures, conformational changes, and molecular interactions. The amide I and amide II bands, located around 1650 cm-1 and 1550 cm-1, respectively, provide information about the protein's backbone conformation. Additionally, IR spectroscopy can be used to monitor protein-ligand interactions, protein folding, and aggregation phenomena.
What are the advantages of ATR-IR spectroscopy over transmission IR spectroscopy?
+ATR-IR spectroscopy offers several advantages over transmission IR spectroscopy, including: reduced scattering effects, minimal sample preparation requirements, and the ability to analyze solids, liquids, and pastes. ATR-IR also enables the analysis of samples with rough surfaces or irregular shapes, making it a versatile technique for various applications.
In conclusion, IR spectroscopy is a powerful and versatile technique that has revolutionized the field of analytical chemistry. Its ability to provide unique molecular fingerprints, combined with its non-destructive nature and high sensitivity, has made it an indispensable tool in various scientific disciplines. As technology continues to advance, we can expect IR spectroscopy to play an increasingly important role in addressing complex chemical challenges and driving innovation across multiple fields.