Multiple Alleles
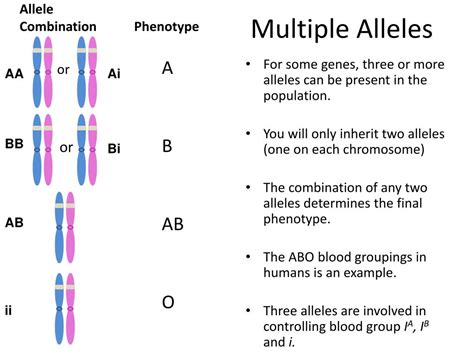
In the intricate tapestry of genetics, the concept of multiple alleles adds a layer of complexity that goes beyond the simple dominance and recessiveness we often associate with Mendelian inheritance. While most high school biology courses introduce the idea of alleles as alternative forms of a gene, the reality is far more nuanced. Multiple alleles occur when a gene exists in three or more allelic forms within a population, influencing traits in ways that defy simplistic explanations. This phenomenon is not just a theoretical curiosity; it underpins many of the genetic variations we observe in nature, from blood types in humans to coat colors in animals. Understanding multiple alleles is crucial for grasping the diversity of life and has profound implications in fields like medicine, agriculture, and evolutionary biology.
The Basics of Multiple Alleles
To appreciate the significance of multiple alleles, let’s start with a foundational concept: alleles are different versions of the same gene that arise through mutation. In the classic Mendelian model, a gene has two alleles (one inherited from each parent), and their interaction determines the phenotype. However, multiple alleles introduce a spectrum of possibilities. For instance, the human ABO blood group system is a classic example of multiple alleles. Here, three alleles—A, B, and O—determine blood type, with A and B being codominant and O recessive.
Mechanisms and Examples
Multiple alleles operate through several genetic mechanisms, including codominance, incomplete dominance, and epistasis. Let’s explore these through real-world examples:
ABO Blood Group System
- Alleles: A, B, O
- Inheritance: A and B are codominant, meaning if an individual inherits both A and B, they express both antigens (type AB). O is recessive, so individuals with OO or AO/BO combinations express type A or B, respectively.
- Implications: Blood type is critical in medicine, particularly for transfusions and organ transplants, where compatibility is essential.
- Alleles: A, B, O
Coat Color in Rabbits
- Alleles: C (full color), c^ch (chinchilla), c^h (Himalayan), c (albino)
- Inheritance: These alleles exhibit a hierarchy of dominance, with C > c^ch > c^h > c. For instance, a rabbit with the genotype Cc^h will have full color, while one with c^hc will be Himalayan.
- Implications: This system illustrates how multiple alleles can create a spectrum of phenotypes, even within a single trait.
- Alleles: C (full color), c^ch (chinchilla), c^h (Himalayan), c (albino)
Sickle Cell Anemia
- Alleles: A (normal hemoglobin), S (sickle cell)
- Inheritance: This is a case of codominance with a trade-off. While AS individuals are resistant to malaria, they can also experience mild sickle cell symptoms. SS individuals have severe sickle cell anemia.
- Implications: This example highlights the evolutionary advantage of multiple alleles, where a harmful allele (S) persists in populations due to its protective effect against malaria.
- Alleles: A (normal hemoglobin), S (sickle cell)
Trait | Alleles | Phenotypes | Significance |
---|---|---|---|
ABO Blood Group | A, B, O | A, B, AB, O | Medical compatibility |
Rabbit Coat Color | C, c^ch, c^h, c | Full color, chinchilla, Himalayan, albino | Phenotypic diversity |
Sickle Cell Anemia | A, S | Normal, carrier, sickle cell | Evolutionary trade-off |

Evolutionary Significance
Multiple alleles are not just genetic curiosities; they play a pivotal role in evolution. By increasing genetic diversity, they provide populations with a broader range of traits to adapt to changing environments. For example, the persistence of the sickle cell allele in malaria-prone regions demonstrates how natural selection can favor alleles that, while harmful in homozygous form, offer a survival advantage in heterozygotes.
Applications in Modern Science
The study of multiple alleles has far-reaching applications:
- Medicine: Understanding multiple alleles helps in diagnosing genetic disorders and predicting disease susceptibility. For instance, knowing an individual’s ABO blood type is crucial for safe transfusions.
- Agriculture: Breeders use knowledge of multiple alleles to develop crops and livestock with desirable traits, such as disease resistance or higher yields.
- Forensics: DNA analysis often relies on multiple allele systems, such as those found in short tandem repeats (STRs), to identify individuals with high precision.
Challenges and Limitations
While multiple alleles offer tremendous insights, their complexity poses challenges. Predicting phenotypes in systems with many alleles and intricate dominance hierarchies can be difficult. Additionally, the interplay between multiple alleles and environmental factors adds another layer of complexity, making it harder to disentangle genetic effects from external influences.
Future Directions
Advances in genomics and bioinformatics are revolutionizing our understanding of multiple alleles. Techniques like whole-genome sequencing and genome-wide association studies (GWAS) allow researchers to identify and analyze multiple alleles with unprecedented precision. This promises to uncover new genetic variants associated with traits and diseases, paving the way for personalized medicine and targeted therapies.
FAQs
What is the difference between multiple alleles and polygenic inheritance?
+Multiple alleles involve three or more variants of a single gene, while polygenic inheritance refers to traits controlled by multiple genes, each contributing a small effect. For example, blood type is determined by multiple alleles at one locus, whereas height is polygenic, influenced by many genes across the genome.
Can multiple alleles exist in all species?
+Yes, multiple alleles can exist in any species with a large enough population size and sufficient genetic diversity. However, their prevalence and impact vary widely across species.
How do multiple alleles contribute to genetic disorders?
+Multiple alleles can lead to disorders when certain combinations result in harmful phenotypes. For example, cystic fibrosis is caused by mutations in the CFTR gene, but the severity can vary depending on the specific alleles involved.
Why are multiple alleles important in evolutionary biology?
+Multiple alleles increase genetic diversity, providing populations with a wider range of traits to adapt to environmental changes. This diversity is essential for long-term survival and evolution.
Can multiple alleles be artificially manipulated?
+Yes, techniques like CRISPR gene editing allow scientists to manipulate specific alleles. This has applications in agriculture, medicine, and biotechnology, but ethical considerations must be addressed.
Conclusion
Multiple alleles are a testament to the elegance and complexity of genetics. They challenge our understanding of inheritance, highlight the interplay between genetics and environment, and underscore the importance of diversity in biology. From blood types to evolutionary adaptations, multiple alleles are a cornerstone of modern genetics, offering insights that extend far beyond the classroom. As we continue to unravel the genetic code, the study of multiple alleles will undoubtedly remain at the forefront of scientific discovery, shaping our understanding of life itself.